What Causes Quantum Tunneling 2025
What Causes Quantum Tunneling 2025
Quantum tunneling is one of the most bizarre and fascinating phenomena in modern physics. Imagine a tiny particle breaking the rules of classical physics—passing through a barrier it seemingly shouldn’t be able to cross. That’s quantum tunneling. In 2025, advancements in quantum field theory and nanoscale technologies have deepened our understanding of this phenomenon and its implications for both fundamental science and practical technologies.
Let’s break down what causes quantum tunneling, how it works, and why it matters.
Book-Level Explanation
In classical physics, if a particle does not have enough energy to overcome a barrier, it simply cannot cross it. For example, a ball thrown at a hill that’s too high will bounce back. But in quantum mechanics, particles behave not just like solid objects but also like waves. This duality is at the heart of quantum tunneling.
Wavefunction and Probability
In quantum mechanics, the behavior of particles is described by a wavefunction (Ψ), which contains information about the probabilities of a particle’s position and momentum. This wavefunction does not abruptly stop at a barrier—instead, it exponentially decays within the barrier. If the barrier is thin or low enough, the wavefunction may still exist on the other side, indicating a non-zero probability that the particle will appear beyond the barrier, even though it doesn’t have the classical energy to cross it.
This is quantum tunneling.
Key Factors Causing Quantum Tunneling:
- Heisenberg’s Uncertainty Principle: This principle states that one cannot precisely know both the position and momentum of a particle simultaneously. This uncertainty allows for small probabilities where a particle may momentarily “borrow” energy to overcome a barrier.
- Wavefunction Penetration: The wavefunction associated with a particle doesn’t just vanish at the edge of a barrier. It decreases but continues through the barrier, leading to a probability that the particle can appear on the other side.
- Barrier Characteristics: The thickness and height of the potential barrier affect tunneling probability. A thinner or lower barrier increases the chance of tunneling.
- Quantum Superposition: A particle doesn’t take a single path; it explores all possible paths simultaneously. This includes paths that involve penetrating a barrier.
Mathematical Expression
In simple cases, the tunneling probability (T) can be approximated as:
T ≈ e^(-2κa)
Where:
- κ = √(2m(V − E)) / ħ
- m is the particle’s mass
- V is the barrier height
- E is the particle’s energy
- a is the barrier width
- ħ is the reduced Planck constant
This formula shows that tunneling is more likely when the barrier is thin, or the energy difference (V − E) is small.
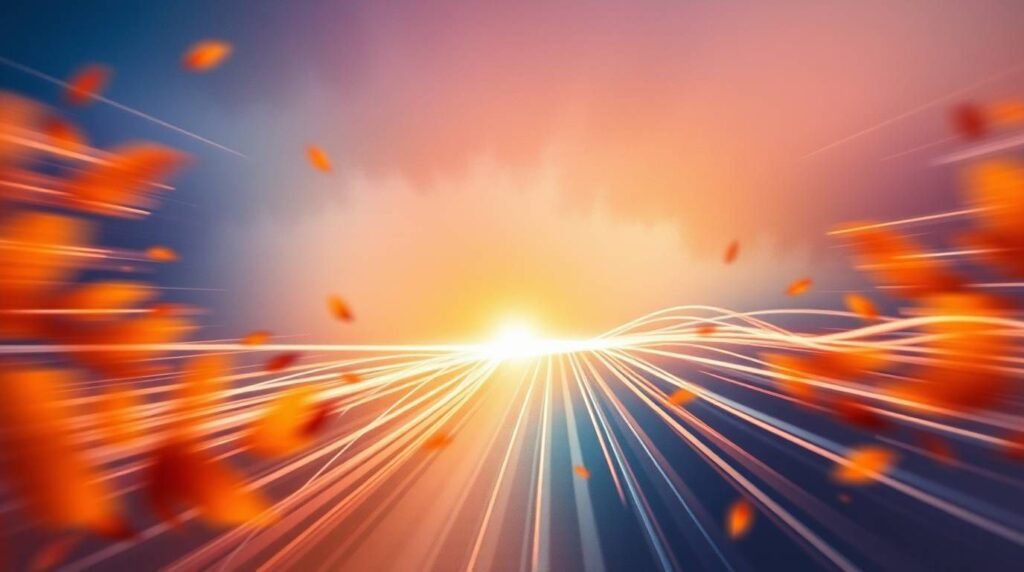
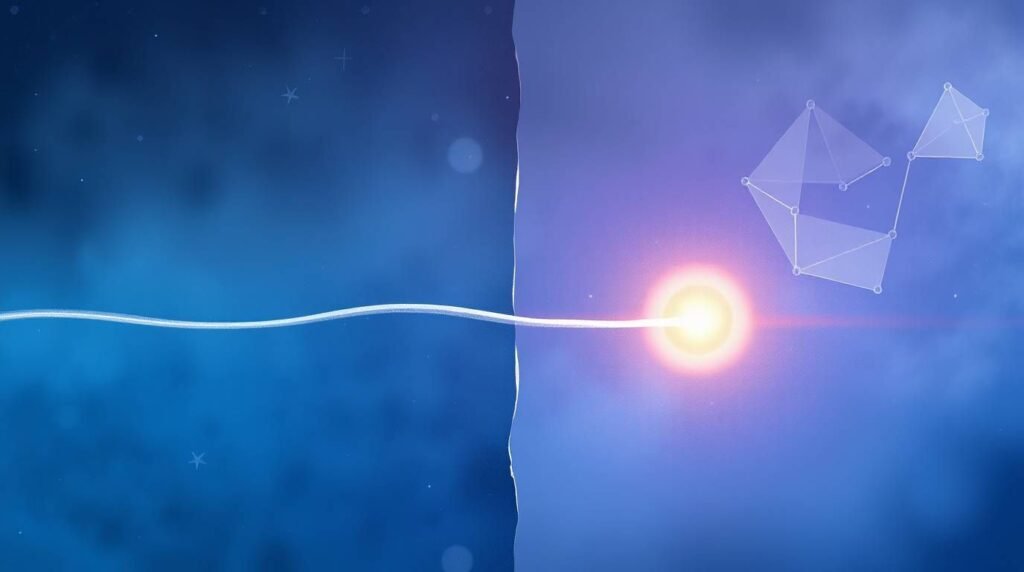
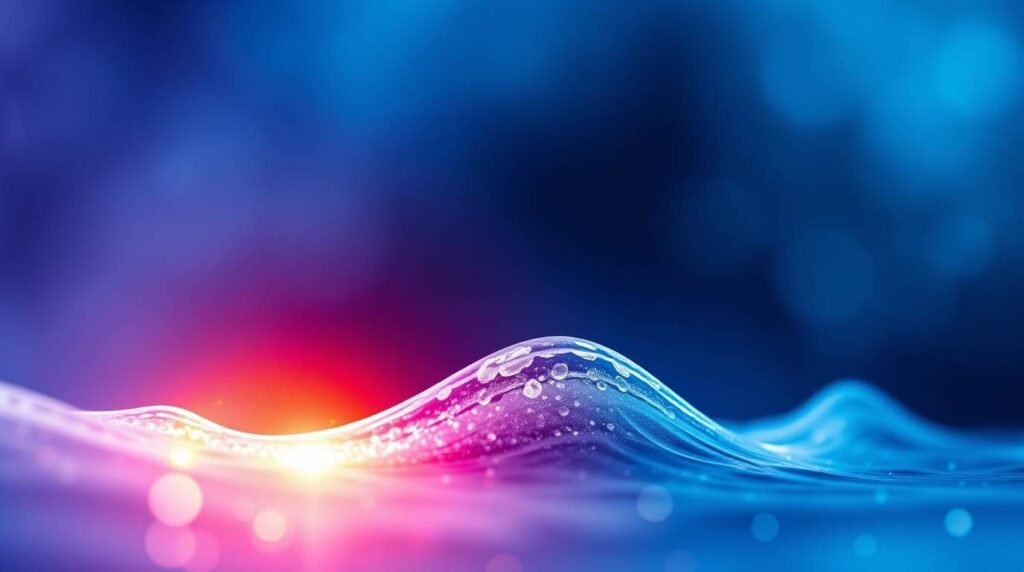
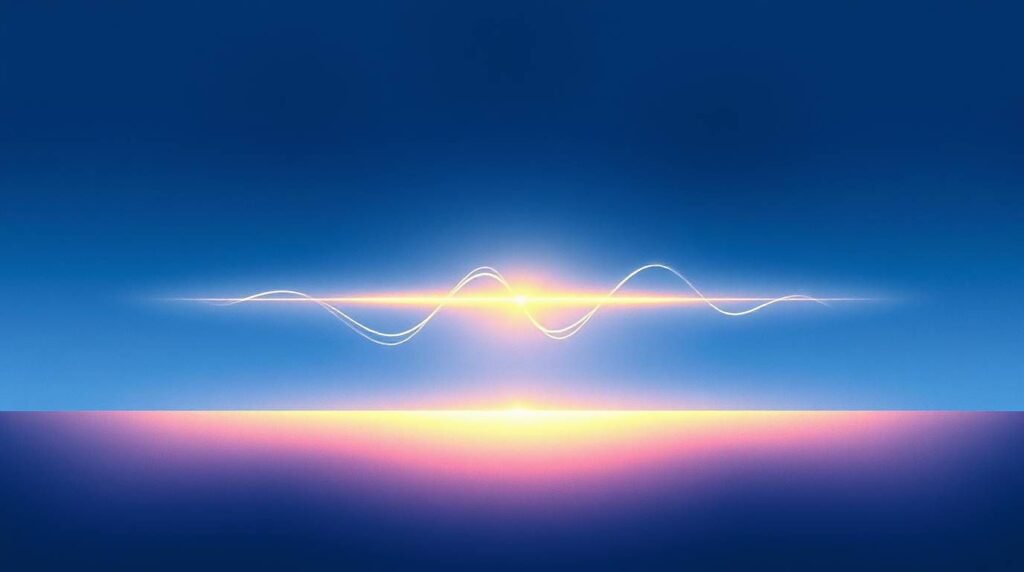
Easy Explanation
Let’s say you’re in a room with walls, and you don’t have the energy to jump over or break through them. In the classical world, you’re stuck. But in the quantum world, you’re like a ghost with a small chance of magically showing up on the other side—even though you didn’t go over or through the wall the normal way.
That’s quantum tunneling.
Particles like electrons are not just little balls—they’re also waves of possibility. These waves can “leak” through barriers. If the barrier isn’t too thick or too strong, the wave goes through a bit, and sometimes the particle appears on the other side.
This doesn’t mean it breaks the rules—it follows the strange rules of quantum mechanics where “impossible” things are just very, very unlikely… but not impossible.
Real-World Applications in 2025
Quantum tunneling isn’t just a curious theory—it powers technologies we use today and is central to cutting-edge research in 2025:
- Semiconductors and Transistors: In modern electronics, especially in nanoscale transistors, tunneling can cause current leakage. Engineers now design devices that either reduce unwanted tunneling or exploit it.
- Tunnel Diodes: These components intentionally use tunneling to allow current to pass through them in unique ways, enabling fast switching electronics.
- Scanning Tunneling Microscope (STM): This device uses tunneling to create atomic-scale images of surfaces. It works by bringing a sharp tip close to a surface and measuring the tunneling current between them.
- Nuclear Fusion and Radioactive Decay: In stars, particles tunnel through barriers to fuse and release energy. Alpha decay in unstable atoms is also caused by quantum tunneling.
- Quantum Computing: Tunneling is a key aspect of quantum bits (qubits) and how they behave inside superconducting quantum circuits.
Why This Matters
Understanding quantum tunneling helps scientists and engineers unlock the potential of quantum mechanics for both theoretical advancements and practical technologies. In 2025, it plays a pivotal role in:
- Quantum chip design for faster processors.
- Quantum sensors with extreme precision.
- Energy solutions, especially in fusion research and superconductivity.
Tunneling shows us how reality works on the tiniest scale—and reminds us that the universe is far more flexible and strange than our everyday experiences suggest.
External Link for Further Reading:
For a deeper dive into the physics of tunneling:
https://en.wikipedia.org/wiki/Quantum_tunnelling
Our Blogs You Might Like
- What If Time Travel Became Scientifically Possible 2025
https://edgythoughts.com/what-if-time-travel-became-scientifically-possible-2025 - How Does Quantum Entanglement Work 2025
https://edgythoughts.com/how-does-quantum-entanglement-work-2025
Disclaimer:
The easy explanation is provided to make the concept accessible to everyone, including beginners. If you’re a student preparing for exams, always refer to your official textbooks, class notes, and follow academic guidelines. Our goal is to help you understand—not replace formal learning.
What If We Could Live Inside a Black Hole? 2025
https://edgythoughts.com/what-if-we-could-live-inside-a-black-hole-2025/
Why can’t objects reach the speed of light?
https://edgythoughts.com/why-cant-objects-reach-the-speed-of-light/